Ameliorative effects of propolis upon reproductive toxicity in males
Article information
Abstract
Propolis is a sticky natural product produced by honeybees. Research studies have discussed the effectiveness of propolis, directly or indirectly, for ameliorating reproductive toxicity in males; however, this research has not yet been reviewed. The current paper presents an integrative summary of all research studies in Scopus and PubMed that investigated the effects of propolis on semen quality, and hence on male fertility, in conditions of reproductive toxicity. The consensus indicates that propolis ameliorates reproductive toxicity and enhances semen quality in vivo in test animals. These effects may be attributable to the ability of propolis to reduce testicular oxidative damage, enhance testicular antioxidant defense mechanisms, increase nitric oxide production, reduce testicular apoptotic injury, and boost testosterone production. However, to generalize these effects in humans would require further research.
Introduction
Propolis, also called bee glue, is a resinous dark-brown mixture produced by honeybees, generally by mixing beeswax and bee saliva with exudates collected from sap flows, tree buds, or other botanic sources [1,2]. Since 1945, particularly in the Scopus database, approximately 7000 published research articles on the impact of propolis on human health have been published, forming a scientific consensus that propolis has potential pharmacological properties. The number of publications on the health effects of propolis has increased dramatically in the last decade. As a result, propolis has been recognized as having antioxidant [3-6], anticancer [7-10], anti-inflammatory [11-13], and antimicrobial and antiviral activities [4,5,14,15]. It has also been recognized as an effective natural product in various diseases and disorders such as cardiovascular diseases [16,17], skin injury and skin care [18,19], diabetes [20-22], neuronal degenerative diseases [23-25], and hypertension [26-28].
Amid the recent increase in research on the ability of propolis to ameliorate specific diseases studies have also investigated the effectiveness of propolis in counteracting reproductive toxicity in males. To date, the PubMed database contains 26 articles that associate propolis and male reproductive toxicity. The current paper reviews these studies and presents an integrative summary of this specific research context. To achieve this collective summary, we gathered studies from Scopus and PubMed that are published in English and that investigate the effects of propolis on male reproductive toxicity. To extract all related studies, we used the keywords “propolis” in combination with “sperm” and “semen.” In addition, to incorporate an explanatory summary of how propolis may function in conditions of reproductive toxicity, relevant mechanistic studies were discussed and reviewed.
Effect of propolis on semen quality in induced reproductive toxicity conditions
Table 1 shows the direct research studies that investigate and discuss the ameliorative effect of propolis against various reproductive toxicants in males [29-38]. These studies were conducted in the last two decades, during which attention toward natural medicine has dramatically increased worldwide [39], and the majority were performed in the Middle East (Egypt and Turkey). One reason for this trend may be that many cohorts in the Middle East are relying on natural remedies [40,41].
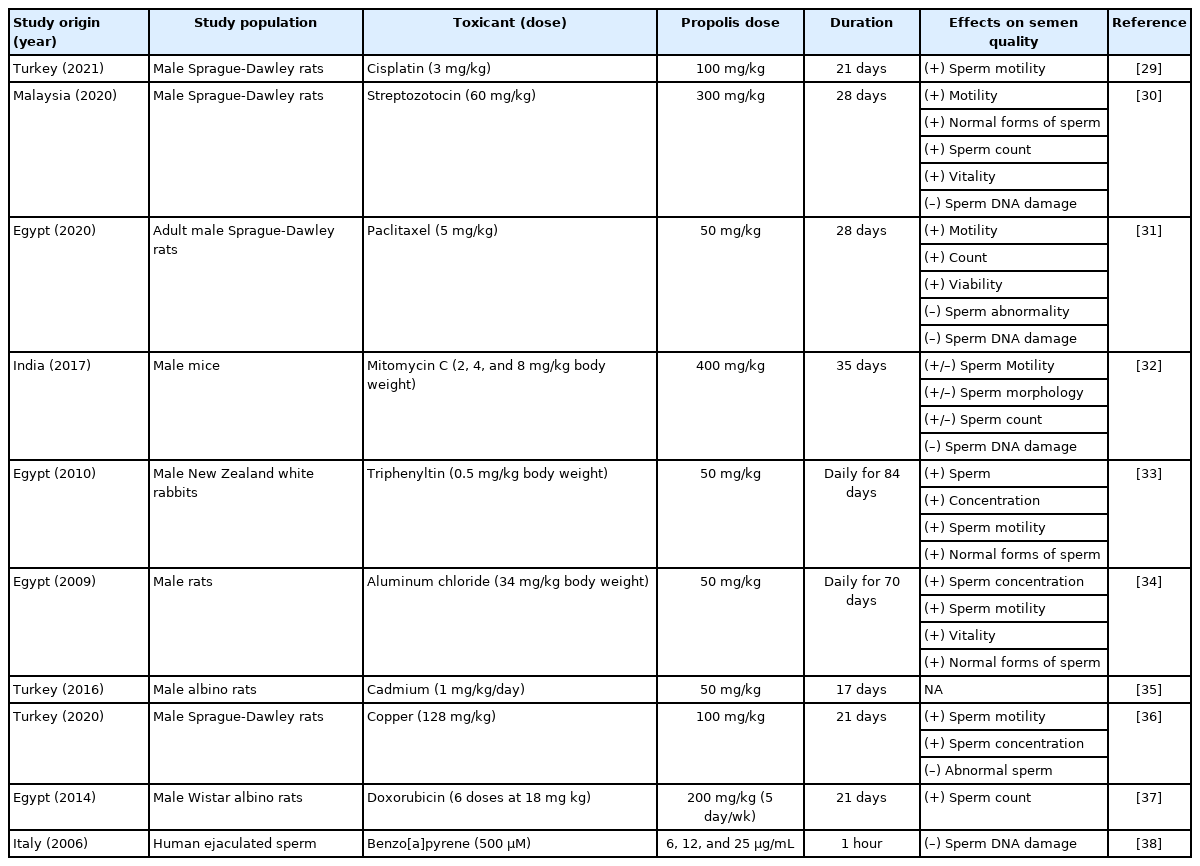
Direct research studies on propolis and its reported effects on semen quality parameters in different conditions of reproductive toxicity induced in males
Almost all included studies that discussed the ameliorative effect of propolis against reproductive toxicants were conducted on animals. The one exception, an in vitro study, was conducted on ejaculated human semen (Table 1). Half of the reproductive toxicants in these studies were chosen to be anticancer agents (cisplatin, paclitaxel, mitomycin C, doxorubicin, and streptozotocin), three were toxic metals (aluminum, cadmium, and copper); one was an anti-fungal agent (triphenyltin), and one was a cancerous agent (benzo[a]pyrene). In in vivo system studies, the duration of exposure to these toxicants ranged from 17 to 84 days, while the propolis dose ranged from 200 mg/kg of body weight (BW), equivalent to approximately 33.3 mg/kg of human BW, to 400 mg/kg of BW, equivalent to 66.7 mg/kg of human BW [42].
Together, almost all included studies revealed protective effects against induced reproductive toxicity (Table 1). In the animals tested, propolis improved semen quality measures such as sperm motility (six studies), sperm quantity (six studies), and normal forms of sperm (four studies). Among the published studies, only one, conducted in India on male mice, revealed a blunted effect of propolis on semen quality measures against mitomycin C-induced reproductive toxicity.
Collectively, these studies form a consensus that propolis may enhance semen quality in vivo when specific reproductive toxicity conditions are induced. However, such effects cannot be generalized to humans without further research.
Mechanisms of action of propolis in ameliorating reproductive toxicity
Gonadal hormones in males, especially testosterone and luteinizing hormone, are considered the primary indicators of sensitivity to exogenous reproductive toxicants. As a result, reducing induced reproductive toxicity by supporting production of these hormones, particularly testosterone, is an important way to measure the bioactivity of a given natural or synthetic substance, due to the hormones’ role in controlling spermatogenesis in males. Briefly, testosterone, which is a steroid hormone chemically derived from cholesterol, is produced in Leydig cells in response to stimulation by luteinizing hormone [43,44], a peptide hormone released from the pituitary gland. It diffuses into Sertoli cells in the seminiferous tubules in the testis [45]. Then testosterone binds to androgen receptors in the cytoplasm and nucleus of Sertoli cells, and triggers the cellular responses needed to support spermatogenesis [45].
Several studies have tested the ability of propolis to counteract a reduction in testosterone induced by exogenous toxicants [33-35,46]. Certain bioactive compounds in propolis, such as the flavonoid chrysin, were found to boost testosterone production in the testes [47]. At the molecular level, propolis was found to enhance steroidogenesis in the testes of diabetic rats by up-regulating the mRNA as well as protein levels of steroidogenic acute regulatory protein (StAR), cytochrome P450 A1 (CYP11A1; an enzyme that converts cholesterol to pregnenolone), cytochrome P450 17A1 (CYP17A1), 3β-hydroxysteroid dehydrogenase (3β-HSD), and 17β-hydroxysteroid dehydrogenase (17β-HSD) [30]. Therefore, the amelioration in semen quality parameters under conditions of reproductive toxicity may be due to the increased levels of testosterone.
Propolis extracts have been identified as having a strong antioxidant activity [48-50]. In vitro investigations revealed that the antioxidant power of propolis is attributable to the existence of many bioactive compounds, particularly phenolic compounds such as flavonoids (e.g., tectochrysin, chrysin, pinocembrin, galangin, apigenin, genkwanin, and kaempferol [51]), stilbenes, flavan-3-ols (catechins), and hydroxybenzoic acids [52]. Additional important phenolic compounds in propolis are cinnamic acid and its derivatives (e.g., hydroxycinnamic acids, ferulic acid, caffeic acid, isoferulic acid, and p-coumaric acid), as well as gallic acid, which have been identified in Polish propolis [53,54].
One known route by which exogenous toxicants induce reproductive toxicity in males is by reducing the activity of testicular antioxidant defense mechanisms. Testicular glutathione (GSH) as well as the testicular antioxidant enzymes glutathione peroxidase, catalase, and superoxide dismutase are often used to measure reproductive toxicity. As Table 2 shows, propolis was able to counteract the induced reduction in antioxidant defense mechanisms by enhancing GSH and antioxidant enzymes in the testis [29-38,46,55]. Given that a reduction in antioxidant defense mechanisms is associated with a decrease in semen quality [56,57], it is possible to infer that the favorable antioxidant effects of propolis may support its ability to improve semen quality when reproductive toxicity is induced.
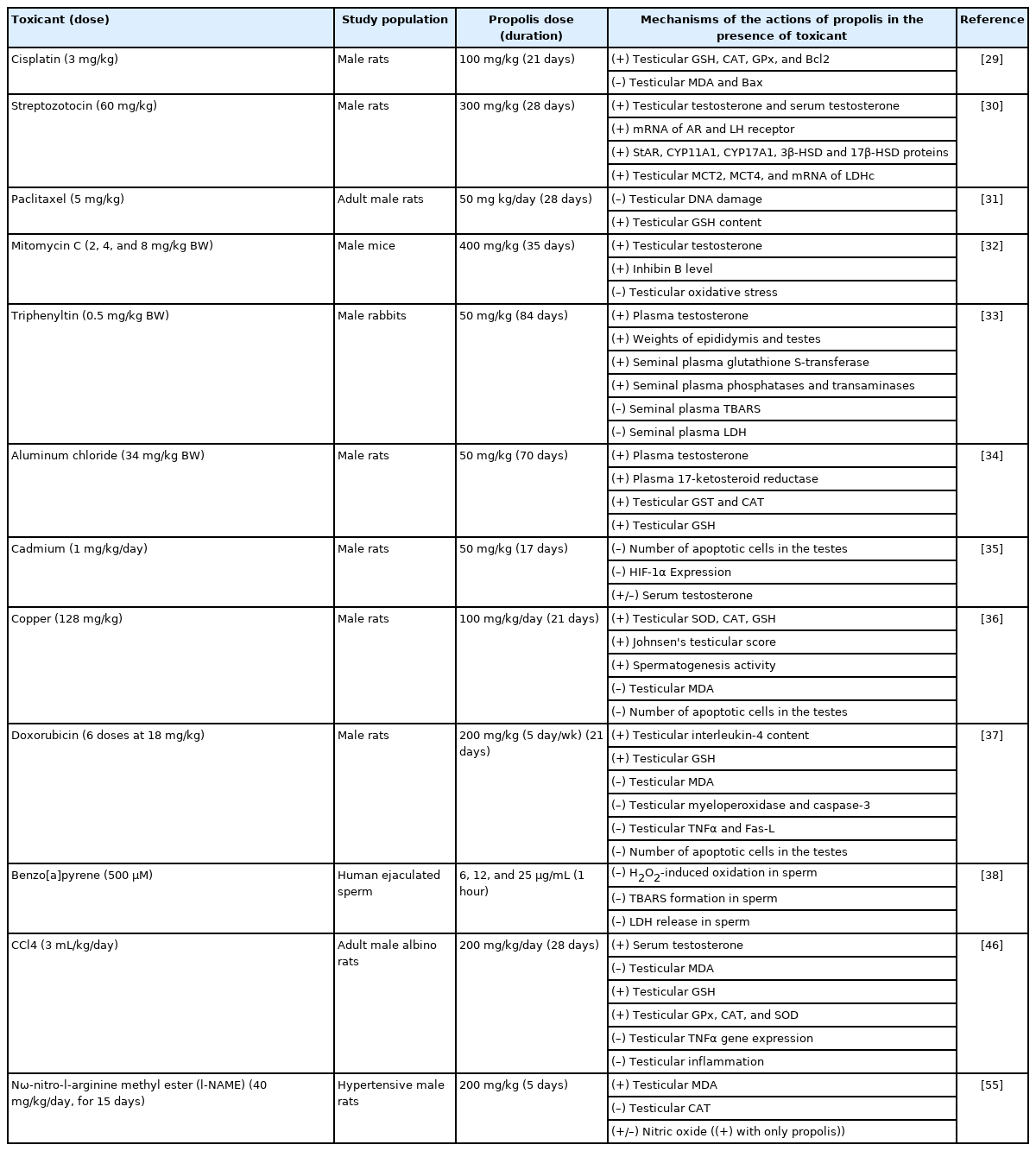
Mechanistic studies on the effect of propolis in conditions of induced reproductive toxicity utilizing different toxicants
Malonyl dialdehyde (MDA), which is a stable end product created from peroxidation of essential (i.e., polyunsaturated) fatty acids in the cell [58], is considered a marker of increased cellular oxidation reactions beyond the capacity of antioxidant defense mechanisms, a state termed oxidative stress [59-62]. Among 12 mechanistic studies, five have revealed a reduction in testicular MDA when propolis is present amid induced toxicity conditions (Table 2). This scientific accord confirms the ability of propolis to protect against induced testicular oxidative damage by exogenous chemical toxicants.
One measure of reproductive toxicity is apoptosis in testicular cells. It is mediated by caspases, proteolytic enzymes belonging to the cysteine protease family, which provoke cell death by cleaving specific proteins in the nucleus and cytoplasm. As shown in Table 2, propolis treatment was found to reduce copper- [36], cadmium- [35], and doxorubicin-induced apoptotic cells in the testes [37], specifically by reducing increased levels of caspases, in particular caspase-3, in induced reproductive toxicity conditions [37]. In addition, propolis treatment prevented the immunoreactivity of hypoxia-inducible factor 1-alpha, and its expression in the testes of the propolis-treated group and the control group was found to be similar, compared to the cadmium-induced group [35].
A second useful marker of reproductive toxicity is the activity of testicular lactate dehydrogenase. In germ cells, lactate dehydrogenase, particularly type C, is required for energy (adenosine triphosphate) production [63]. In effect, a decline in the availability of lactate for germ cell development was found to contribute to the impairment of male reproductive function [63,64]. Propolis administration has been shown to enhance the activity of lactate dehydrogenase in conditions of induced reproductive toxicity, such as streptozotocin- and benzo[a]pyrene-induced toxicity [38,30].
Nitric oxide is a gaseous signaling molecule synthesized naturally in the body by nitric oxide synthases [47,65,66]. It acts as a vasodilator, particularly for blood vessels and inner muscles, which consequently increases circulation in these tissues [67]. Nitric oxide has been found to have a crucial role in male reproduction [68,69]. For example, it boosts the blood flow in the testes, thereby enhancing the activity of their physiological processes [70]. One important example is boosting testosterone synthesis by Leydig cells and subsequently activating spermatogenesis in Sertoli cells [71-73]. In male rats, propolis has been found to increase nitric oxide production in testicular tissue [55], which may support spermatogenesis.
In summary, there is evidence that propolis ameliorates reproductive toxicity and improves semen quality in vivo in test animals, but not in humans. Mechanistically, the protective effects of propolis may be due to reducing testicular oxidative damage, enhancing testicular antioxidant defense mechanisms, enhancing production of nitric oxide, reducing testicular apoptosis and cell death, and improving testosterone production.
Notes
Conflict of interest
No potential conflict of interest relevant to this article was reported.