Decorin: a multifunctional proteoglycan involved in oocyte maturation and trophoblast migration
Article information
Abstract
Decorin (DCN) is a proteoglycan belonging to the small leucine-rich proteoglycan family. It is composed of a protein core containing leucine repeats with a glycosaminoglycan chain consisting of either chondroitin sulfate or dermatan sulfate. DCN is a structural component of connective tissues that can bind to type I collagen. It plays a role in the assembly of the extracellular matrix (ECM), and it is related to fibrillogenesis. It can interact with fibronectin, thrombospondin, complement component C1, transforming growth factor (TGF), and epidermal growth factor receptor. Normal DCN expression regulates a wide range of cellular processes, including proliferation, migration, apoptosis, and autophagy, through interactions with various molecules. However, its aberrant expression is associated with oocyte maturation, oocyte quality, and poor extravillous trophoblast invasion of the uterus, which underlies the occurrence of preeclampsia and intrauterine growth restriction. Spatiotemporal hormonal control of successful pregnancy should regulate the concentration and activity of specific proteins such as proteoglycan participating in the ECM remodeling of trophoblastic and uterine cells in fetal membranes and uterus. At the human feto-maternal interface, TGF-β and DCN play crucial roles in the regulation of trophoblast invasion of the uterus. This review summarizes the role of the proteoglycan DCN as an important and multifunctional molecule in the physiological regulation of oocyte maturation and trophoblast migration. This review also shows that recombinant DCN proteins might be useful for substantiating diverse functions in both animal and in vitro models of oogenesis and implantation.
Characteristics and functions of decorin
About 20 years ago, proteoglycan gene families were classified and simplified according to three criteria: their cellular and subcellular location, the overall gene and protein homology, and the presence of specific protein modules within their respective protein cores. In particular, the largest class is formed by a set of extracellular proteoglycans encoded by 25 genes. The first group contains the 4 genes for hyalectan, a major structural component of cartilage, blood vessels, and the central nervous system. The second comprises 18 small leucine-rich proteoglycans (SLRPs). These SLRPs have a multitude of functions, including signaling through various receptors. Many SLRPs are found in the circulation and various body fluids. The third is the SPOCK family of calcium-binding heparan sulfate proteoglycans [1].
The SLRPs expressed in most extracellular matrices (ECMs) generally can act as structural components to maintain tissue architecture. They are involved in range of fundamental biological and physiological functions, including cell adhesion, signal transduction, and the immune response. SLRPs share many biological functions by binding to ECM components, particularly various collagens, receptor tyrosine kinases, and innate immune receptors (Toll-like receptors) on cell surfaces when present in a soluble form [2,3]. They are characterized by a relatively small protein core of 36–42 kDa, including leucine-rich repeats (LRRs) [4]. Individual LRR modules have 20–30 amino acid residues with a highly conserved “LxxLxLxxN” motif. The central LxL part of the module forms the core β strand, with two leucines pointing towards the interior of the protein, making up the hydrophobic core, whereas the variable x residues within the motif are exposed to solvent. Some are involved in interactions with ligands. Aspargines in the motif make continuous hydrogen bonds with backbone carbonyls of neighboring β-strands throughout the entire protein. This extended hydrogen bond network is called the “asparagine ladder.” Therefore, β-strands are more closely packed and assembled into a large β-sheet, making up the entire concave surface of the horseshoe. Variable amino acids, except the conserved β-strands of each LRR module, are surface-exposed. Some of them play important roles in ligand interactions. To prevent the exposure of hydrophobic core of LRR modules, it has two special modules named LRRNT and LRRCT in the N- and C-termini of proteins. These modules do not follow the sequence conservation pattern of LRR modules. They often contain an anti-parallel β-hairpin stabilized by disulfide bridges [5-7].
The 18 SLRP members are grouped into five classes. Classes I–III have the conserved C-terminal cysteine-rich capping motif, a unique feature that has recently been described as the “ear repeat,” appearing as an abnormal pattern of cysteine residues followed by the asparagine residue in consensus sequences. Classes IV and V are noncanonical fragments without the ear repeat [1,2,4]. The sequence alignment and phylogenetic tree of the SLRPs are presented in Figure 1.
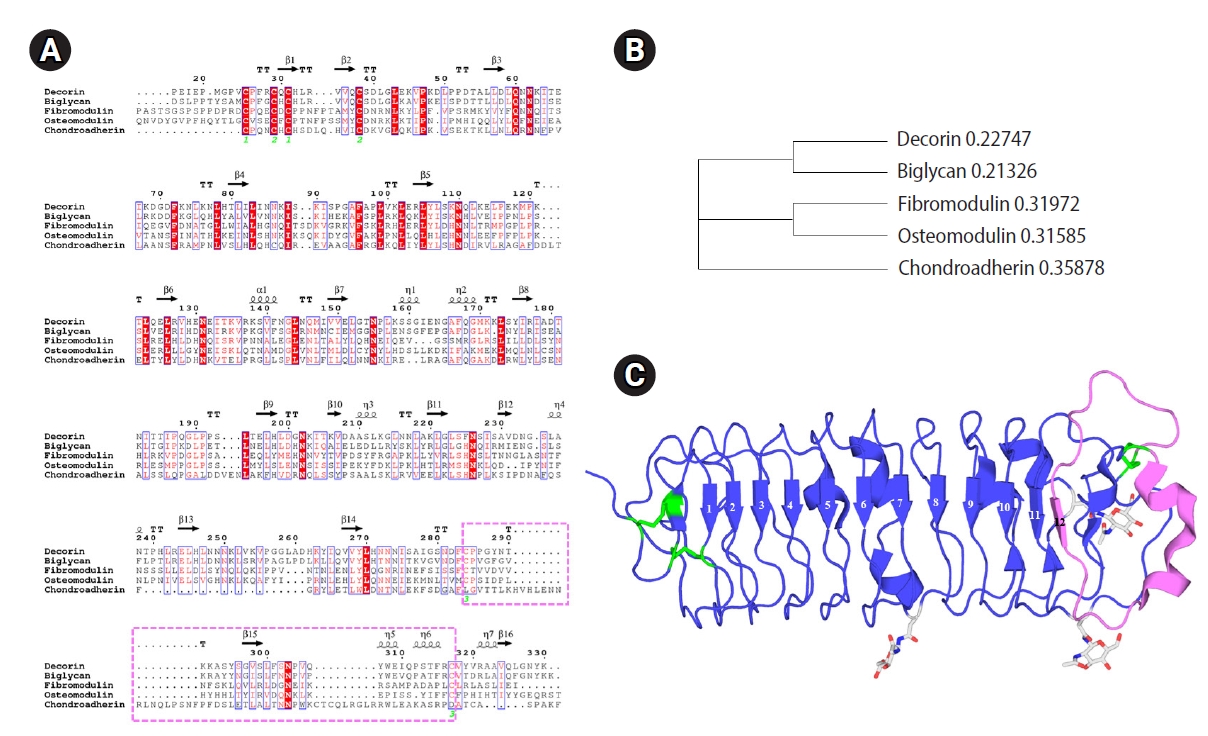
Sequence alignment and phylogenetic tree of small leucine-rich proteoglycans (SLRPs) using known crystal structures of decorin (PDB ID: 1XKU), biglycan (PDB ID: 2FT3), fibromodulin (PDB ID: 5MXO), osteomodulin (PDB ID: 5YQ5), and chondroadherin (PDB ID: 5LFN). (A) Sequence alignment of crystal structures of five SLRPs. Protein sequences were aligned with Clustal Omega (Clustal Omega<Multiple Sequence Alignment<EMBL-EBI) and generated using the EsPript 3.0 program (ESPript 3 [ibcp.fr]). (B) Phylogenetic tree of SLRPs with known crystal structures. (C) Diagram of the crystal structure of Bos taurus decorin rendered with PyMOL v1.8. Vertical arrows indicate β-strands. Coiled ribbons indicate α-helices. Leucine-rich repeats (LRRs) are numbered above the β-strands. N-acetylglucosamine is linked to N182, N233, and N274. Disulfide bridges are shown in green. The terminal LRR Cys capping motif, known as the ear repeat, is highlighted in pink.
Decorin (DCN), which belongs to class I, is produced by a variety of stromal cells in the body, such as fibroblasts in the dermis, cornea, and chondrocytes of cartilage. It may participate in ECM remodeling during the attachment and detachment of the placenta within the course of pregnancy in cows [8]. Those with disrupted DCN genes are viable, but they show fragile skin with markedly reduced tensile strength. As a result, aberrant collagen morphology appears in the skin and tendon with coarser and irregular fiber outlines [9]. Normal DCN expression regulates a wide range of cellular processes including proliferation, migration, apoptosis, and autophagy through interactions with various molecules. However, aberrant expression of DCN has been associated with poor extravillous trophoblast (EVT) invasion of the uterus, which underlies the occurrence of preeclampsia (PE) and intrauterine growth restriction (IUGR) [10].
During pregnancy, placental cells are under tight hormonal control. Among others, they regulate the concentration and activity of specific proteins participating in the ECM remodeling of fetal membranes [11]. Therefore, the proteoglycan DCN plays a variety of roles. The most important role of DCN is that it can regulate cell adhesion mediated by various binding proteins and tissues for fetal formation.
Production of recombinant DCN protein in the laboratory
For functional analysis, DCN should be made by recombinant DNA technology in the laboratory. The whole gene of the human DCN protein was amplified by PCR and cloned into a modified pAcGP67a vector. The cloned DNA of the DCN gene was confirmed by sequencing. Large-scale DNA preparations were then performed to obtain a sufficient amount of transfection-grade plasmid DNA, which was co-transfected with ProGreen (a baculovirus genomic vector) into Sf9 (Spodoptera frugiperda) insect cells. The vector was then generated and amplified by recombination between the cloned vector and viral DNA. The Fc tagged DCN protein was expressed in High Five cells. The culture media of the High Five cells were harvested and supernatants containing secreted DCN-Fc proteins were purified by protein A affinity chromatography. The molecular mass of the DCN protein was calculated to be approximately 37.9 kDa, with an isoelectric point (pI) of 8.61, using the theoretical tool “Compute pI/Mw” (https://web.expasy.org/compute_pi/). The DCN-Fc tag was cleaved by thrombin. Using Superdex 200-size exclusion chromatography (SEC), the cleaved DCN was further purified. It was found to be a monomer, which was validated as a SEC standard component. The purification steps of affinity and SEC were monitored by sodium dodecyl sulfate-polyacrylamide gel electrophoresis (SDS-PAGE). The above procedure in insect cells allowed the efficient generation of recombinant DCN protein, which was subsequently expressed and purified as shown in Figure 2.
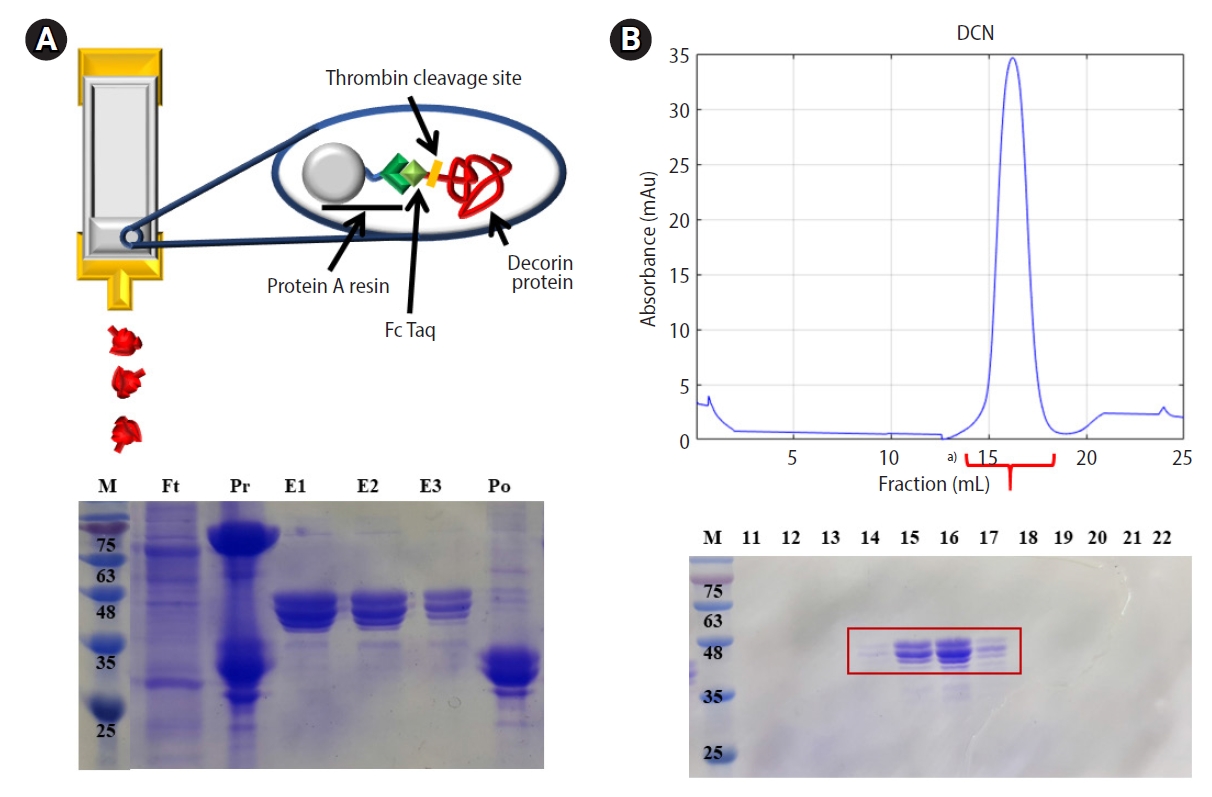
Production of the recombinant decorin (DCN) protein in insect cells. Recombinant human DCN was generated by a modified pAcGP67a vector. It was purified by affinity chromatography and size exclusion chromatography (SEC). (A) Scheme of affinity chromatography using protein A resin and sodium dodecyl sulfate-polyacrylamide gel electrophoresis (SDS-PAGE) gel showing the purity of DCN. Lane 1: marker (M); lane 2: flow-through (Ft); lane 3: pre-resin (Pr), which was DCN-Fc bound protein A resin before thrombin treatment; lanes 4-6: elutions (E1-E3) of DCN after thrombin treatment; lane 7: post-resin (Po), which was the remaining resin after elutions of DCN. (B) Elution profiles of SEC and SDS-PAGE gel showing the purity of DCN. For SDS-PAGE analysis, lane 1: marker (M); lane 2-12: fraction numbers of eluted DCN. a)Indicates peak containing DCN.
DCN in the ovary and oocyte maturation
The ovary in adults has diverse functions, including follicle growth and maturation, ovulation, regression, and corpus luteum (CL) formation. During this process, bidirectional communication between follicles and the ECM may influence the quality of oocytes in mammals. Many studies have demonstrated that DCN is present in the ECM of normal and tumorous ovaries in humans [12,13]. As discussed above, DCN is a secreted proteoglycan that plays a structural role in the ECM. It can interfere with the signaling of multiple growth factors and their receptors [14]. For instance, DCN can directly bind to epidermal growth factor receptor (EGFR), which has many physiological functions in oocyte maturation [15,16]. When DCN binds to EGFR, it causes phosphorylation and activation of EGFR, followed by internalization and downregulation of EGFR signaling, resulting in a chronic blockage of the EGF/EGFR signaling pathway [17-21]. In the ovary, EGF-like molecules, including amphiregulin and epiregulin, with roles in the orchestration of ovulation and subsequent development of the CL in response to the luteinizing hormone surge are produced in a fine-tuned manner [15,22]. Regarding human oocyte maturation, EGF also targets human oocytes to regulate their meiotic maturation [23]. According to a previous report, DCN is present in human and monkey ovarian stroma, follicular theca cells, luteal cells, and the follicular fluid of ovulatory follicles. It has been postulated that DCN can act as a paracrine signaling factor by inhibiting growth factor/growth factor receptor interactions, suggesting that DCN might regulate folliculogenesis and oocyte quality [24]. Peng et al. [25] synthesized full-length cDNA of goat DCN and identified its expression patterns in various tissues and the ovary. They also confirmed that over-expressed DCN not only could promote programmed cell death through a non-mitochondrial apoptosis pathway, but also could enhance cell arrest by p21 upregulation. Notably, the physiological actions of DCN are mediated through multiple signaling pathways, including the PKA, p38 kinase, and PI3K pathways. Kedem et al. [26] recently conducted a prospective study involving 49 patients treated at a local assisted reproductive technology (ART) center. They characterized the in vivo expression of DCN mRNA in mural and cumulus granulosa cells (CGCs). In previous studies, the human chorionic gonadotropin–induced expression of DCN was found to be highly upregulated in pre-ovulatory follicles [27-29]. Interestingly, DCN expression in human CGCs seems to be correlated with maturation stage of the corresponding oocyte. A plausible explanation is that oocytes that arrest at immature stages under controlled ovarian hyperstimulation finally fail to undergo maturation. Their adjacent cumulus cells also fail to express DCN. Another explanation is that downregulated DCN expression in CGCs is one of causative factors for meiotic arrest. Miscommunication between the oocyte and surrounding somatic cells may result in subfertility [26].
Sawada et al. [30] suggested that DCN in the follicular fluid (F-DCN) is a useful biomarker of the quality of oocytes retrieved from the corresponding follicles in ART. They analyzed 130 oocytes of 88 patients treated with ART because of unexplained infertility. In patients with controlled ovarian stimulation protocols, the median level of F-DCN was slightly higher than that in the serum (S-DCN). However, F-DCN showed a weak negative correlation with S-DCN. They suggested that DCN actively taken into the follicular fluid from blood might play beneficial roles in follicle and oocyte maturation. Regardless of the fertilization method, fertilized eggs showed no significant differences between F-DCN and S-DCN. Interestingly, F-DCN of fertilized oocytes was significantly lower than that of unfertilized oocytes only in patients who underwent intracytoplasmic sperm injection. They also proved that the F-DCN level in intracytoplasmic sperm injection patients had potential to predict fertilization success based on a receiver operating characteristic (ROC) curve analysis. When they established a cut-off level of 34.5 ng/mL for F-DCN based on the ROC curve, they suggested that oocytes from follicles with F-DCN lower than the cut-off level tended to be better than those from oocytes with a high F-DCN [30].
DCN in the uterus and trophoblast migration
For successful blastocyst implantation, invasive trophoblast cells should mediate embryonic migration into the decidual matrix, forming abundant networks connecting embryonic tissue to maternal blood vessels. Signaling in pregnancy can induce the differentiation of endometrial stromal cells into decidual cells. Human decidual cells stimulated by steroid hormones can produce 2 SLRPs: DCN and biglycan. The maintenance of pregnancy is guided by the composition and organization of the endometrial ECM in the uterus. Certain pathological conditions that occur during pregnancy, including PE, have been linked to abnormal placental morphology and consequent fetal morbidity. In the uterus of a knockout mouse model, DCN was found to be required for myometrium contraction in a DCN concentration-dependent manner, whereas biglycan exhibited partial compensation for DCN loss [31].
Transforming growth factor (TGF)-β and DCN are produced in the human feto-maternal interface. They play decisive roles in the regulation of trophoblast invasion in the uterus. TGF-β has DCN binding sites, and its activity is controlled by DCN. Lysiak et al. [32] reported that TGF-β and DCN were co-localized in the ECM of first-trimester decidual tissues. They suggested that DCN might inhibit the activity of TGF-β in the ECM of the placenta. In addition, it has been suggested that migration, proliferation, and invasion of EVTs and choriocarcinoma cells are independently regulated by interactions between TGF-β and DCN in decidual tissues [33]. At the human feto-maternal interface, the decidua forms a dense ECM structure that can regulate trophoblast invasion. Experimentally, silencing of KAI1 (a metastasis suppressor) by double-stranded RNA interference reduced expression of DCN, a decidual product implicated in limiting trophoblast invasion. It has been shown that KAI1 is expressed in decidual cells at the feto-maternal interface, where it might participate in the control of trophoblast invasion [34].
Several reports have shown that DCN in decidual tissue could regulate migration, proliferation, and invasion of EVTs of the human placenta in a TGF-β-independent manner [33-35]. These functions were differentially mediated by the binding of DCN to various tyrosine kinase receptors, including IGFR1, EGFR, and VEGFR2 [35]. It has been found that the overexpression of DCN in basal decidual cells is associated with a hypo-invasive phenotype and poor endovascular differentiation of trophoblast cells in PE [36]. Supplementation of DCN and knockdown of c-Met can reduce the proliferation and invasion in HTR-8 trophoblast cells. However, induction of autophagy and apoptosis by DCN were not synergistically enhanced by c-Met knockdown. It was found that DCN could promote autophagy and apoptosis mainly through downregulating c-Met/Akt/mTOR activity in human trophoblast cells [10].
Halari et al. [37] found that DCN production increased during the decidualization of human endometrial stromal cells in vitro and early gestation in decidual samples tested ex vivo. Endometrial stromal cell maturation and differentiation into decidual cells are crucial for a normal pregnancy. In their study, depleting DCN in human endometrial stromal cells treated with decidualization stimulation failed to induce morphologically and functionally appropriate maturation and differentiation of decidual cells [37]. Recently, it has been suggested that 2 SLRPs, DCN and biglycan, play important roles in the structural and functional integrity of the placenta and fetal membrane and that their alterations may lead to several pregnancy-related diseases such as repeated implantation failure, PE, IUGR, and premature rupture of membranes [4].
Conclusion
DCN is a multifunctional molecule. Its functions are mediated by a variety of binding events, including receptor-mediated and glycosaminoglycan-mediated binding. At the human feto-maternal interface, TGF-β and DCN play crucial roles in the regulation of trophoblast invasion in the uterus. TGF-β has DCN binding sites. Its activity is controlled by DCN. During pregnancy, orchestrated hormonal control of successful pregnancy should regulate the concentration and activity of specific proteins such as DCN participating in the ECM remodeling of trophoblastic and uterine cells. This review confirms that proteoglycan DCN is an important and multifunctional molecule in the physiological regulation of oocyte maturation and trophoblast migration. The findings discussed herein suggest that recombinant DCN proteins might be useful for substantiating these diverse functions in both animal and in vitro models of oogenesis and implantation (Figure 3).
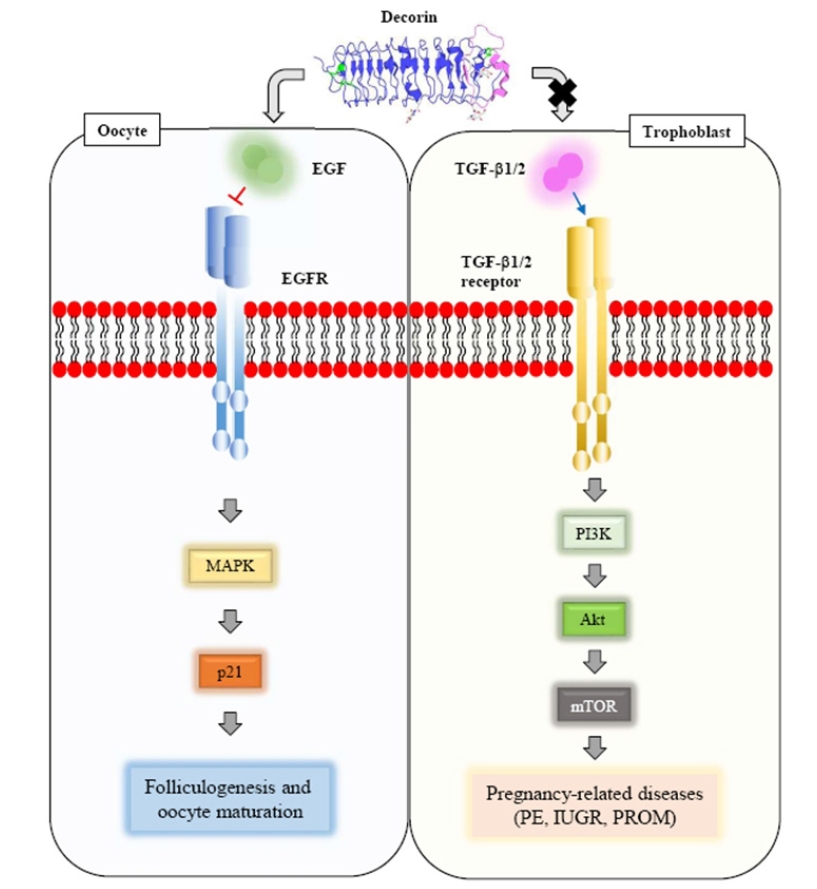
Schematic diagram showing how decorin acts as a multifunctional molecule during oocyte maturation and embryo implantation. EGF, growth factor receptor; EGFR, epidermal growth factor receptor; TGF, transforming growth factor; PE, preeclampsia; IUGR, intrauterine growth restriction; PROM, premature rupture of membranes.
Notes
Conflict of interest
No potential conflict of interest relevant to this article was reported.
Author contributions
Conceptualization: all authors. Data curation: all authors. Formal analysis: all authors. Funding acquisition: BSP. Methodology: all authors. Project administration: all authors. Visualization: all authors. Writing–original draft: all authors. Writing–review & editing: all authors.
Acknowledgements
We thank Ho min Kim (from the IBS and KAIST) for his help with protein expression.